by Femke van Woesik and Frank van Steenbergen
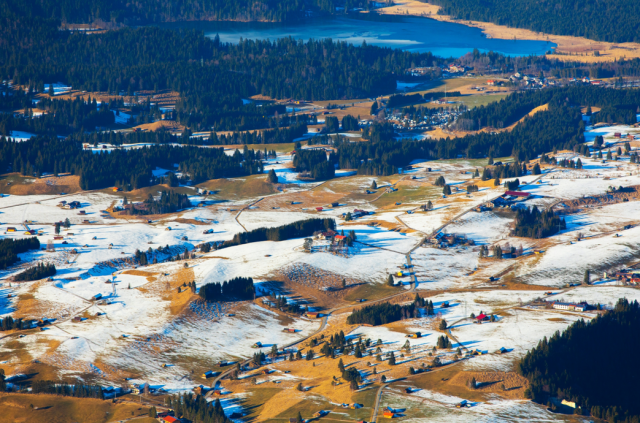
This sight is probably quite familiar for people living in an area with snowy winters. After a snowfall event or during spring, some patches in a landscape retain the snow for a longer time, resulting in a patchwork of different surface coverages over the landscape (Photo 1). How is this possible, and why is there less snow in certain spots? The answer is: microclimates.
Microclimates result from multiple components: solar radiation, soil moisture, soil and air temperature, air humidity, and wind direction and speed. All these components are inextricably linked to each other and work together as a system, forming different microclimates. These microclimatic factors are always at play; however, the patches of snow scattered through landscapes make microclimates more visible. Microclimates reveal themselves during these snowy times and display their high variability in temperature, wind, received solar radiation, and more. This makes snowy landscapes a great opportunity to study microclimates.
For example, when snow falls during stronger winds, some areas are left with thicker or thinner snow cover, depending on the direction and speed of the wind in an area. Wind in a landscape might also blow the snow from the ground and pile this in places in the lee of obstacles or lower spots such as ditches or empty ponds. In certain landscapes, warm winds can also make the snow disappear due to sublimation. Sublimation is the process of changing from a solid to a gas without passing through an intermediate liquid phase. In dry, warm winters, this sublimation process transforms the solid snow into water vapour and carries the water vapour away. This results in some microclimates with less wind able to retain snow cover longer than areas with much wind.
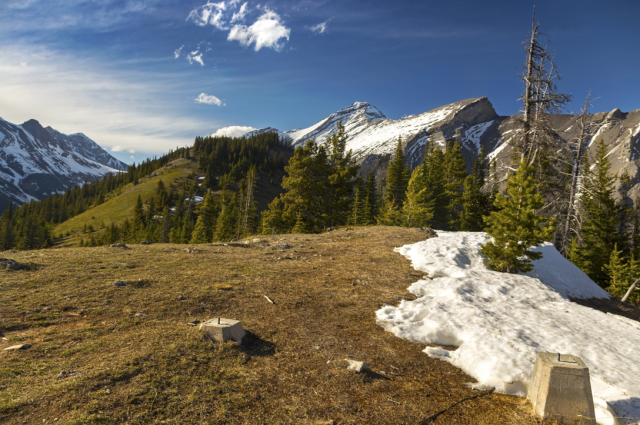
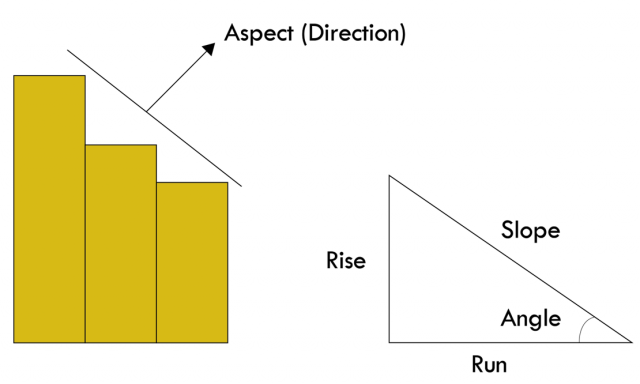
Solar radiation is another significant factor in forming microclimates and affecting snow accumulation and distribution. The amount of solar radiation received by an area is highly dependent on the aspect (compass direction) and slope of that landscape (steepness of angle with the horizontal plane) (figure 1). A hillslope facing north in the northern hemisphere may retain snow much longer than a hillslope facing toward the south (photo 3).
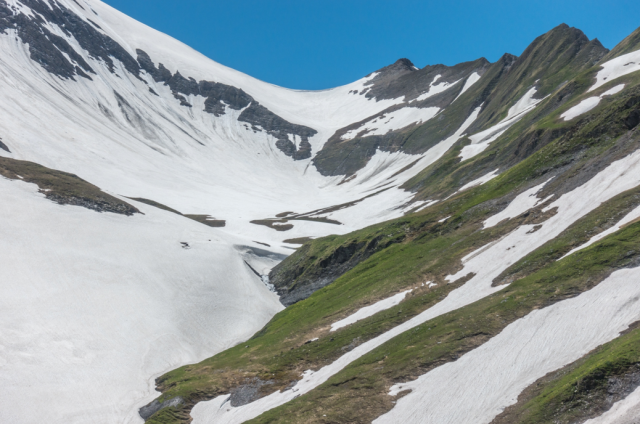
Solar radiation may also heat objects such as trees, buildings, or rocks, radiating heat throughout the night, making the snow melt faster (photo 4) and revealing the warmer microclimates around them. However, in colder places, trees can make snow lasts longer by protecting it from sun and wind.
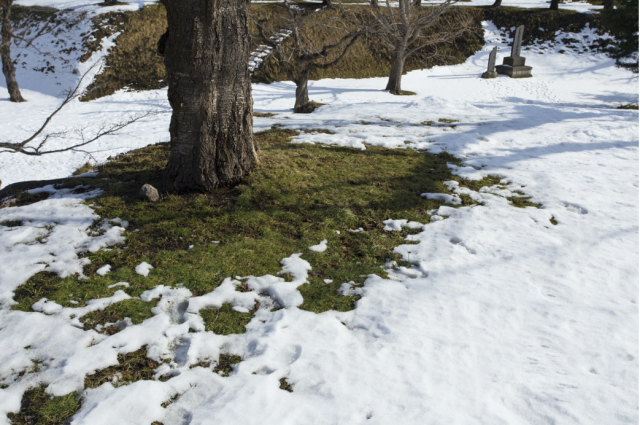
Next to wind and solar radiation, the soil temperature is another microclimate component that highly influences snow accumulation and melting rates. The soil needs to be cold for the snow to accumulate and not melt away immediately. The soil temperature results from incoming solar radiation, the more solar radiation is absorbed by the soil, the higher its temperature. How much solar radiation is absorbed and how much solar radiation gets reflected is determined by the albedo of the surface (Figure 2). A cover with a high albedo (light-coloured) reflects a lot of the incoming sunlight, and a surface with a low albedo (darker colour) absorbs a lot of the incoming sunlight. The lower the albedo level and the higher the soil temperature, the more likely it is that the snow will melt faster.
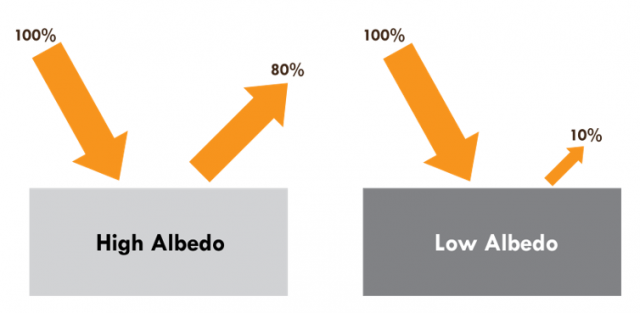
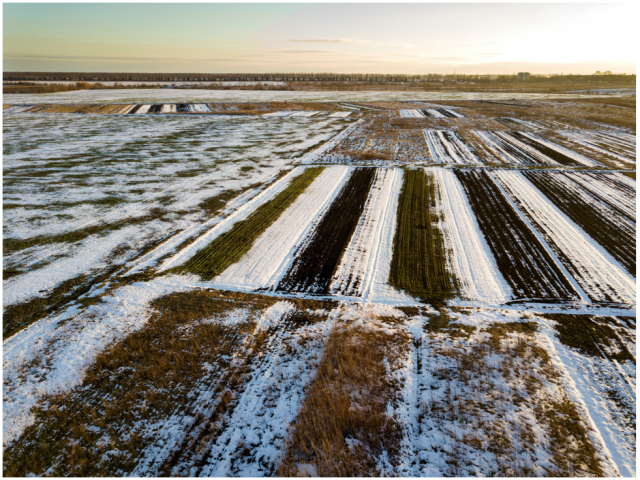
Farm fields can also show high snow cover variation because of agronomic practices such as tillage. Reduced tillage and no-tillage (conservation tillage) cause a greater snow accumulation[1]. This is because on conventionally tilled fields, snow just blows off ridge tops, and then it accumulates as large drifts on north slopes and bottomlands. In a conservation tillage system, the residue left on the field significantly increases snow cover amount and uniformity across the field[2]. These differences in soil temperature and soil structure thus result in a patchwork of snow cover consisting of fully covered areas interchanged by bare patches (Photo 5 & Photo 6).
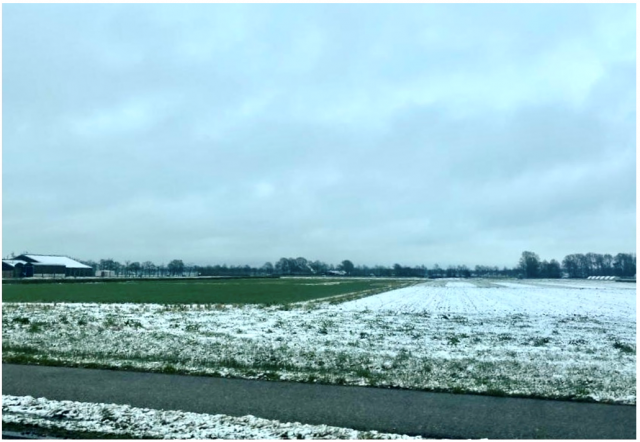
What does this imply for farming? Microclimates where snow retains longer can be highly valuable to crop growth as snow cover has considerable temperature-regulating properties, essential for agricultural soils. The high porosity and air content of snow make it an excellent insulator that maintains above-freezing temperatures in soils even when air temperatures above the snowpack are below 0 °C[1]. Without snow cover, low temperatures can directly affect the soil and result in deep freezing of the soil profile, changing the soil’s hydraulic properties dramatically[2]. The snow cover also protects plants from exposure to desiccating winds and can increase soil water recharge rates after melting.
As mentioned above, microclimatic factors are always at play. However, snowy winters and springs make them visible and can help us identify different microclimates in a landscape. On a small plot scale, the snow distribution can reveal cold traps that longer retain snow, and more heated areas were snow melts faster. According to this, one can evaluate the plot for the best growing spots for specific plants. On a larger scale, taking note of snow patterns gives valuable insights into the workings of landscapes.
[1] Decker, K.L., Wang, M.D., Waite, C. & Scherbatskoy, T. (2003) Snow removal and ambient air temperature effects on forest soil temperatures in Northern Vermont. Soil Science Society of America Journal, 67, 1234–1243.
[2] Bechmann, M. E., & Bøe, F. (2021). Soil tillage and crop growth effects on surface and subsurface runoff, loss of soil, phosphorus and nitrogen in a cold climate. Land, 10(1), 77.
[1] Benoit, G. R., Mostaghimi, S., Young, R. A., & Lindstrom, M. J. (1986). Tillage-residue effects on snow cover, soil water, temperature and frost. Transactions of the ASAE, 29(2), 473-0479.
[2] Perry, A. (2012). No-till and snow can help crops grow. Agricultural Research, 60(7), 8.